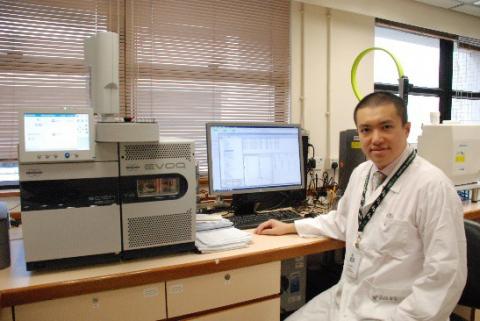
Biochemical genetics in the expanded newborn screening era
Volume 14, Issue 1 January 2019 (download full article in pdf)
Editorial note
In this topical update, Dr. Calvin Chong reviews and updates on the technological development of biochemical genetics for the three major classes of metabolic disorders, namely aminoacidopathies, organic acidurias and fatty acid oxidation defects. These conditions have increasing local awareness, in particular with the introduction of universal expanded newborn screening. With a rising clinical demand for confirmatory tests in biochemical genetics, the ways to achieve better analytical quality and capacity were discussed. We welcome any feedback or suggestions. Please direct them to Dr. Sammy Chen (email: chenpls@ha.org.hk) of Education Committee, the Hong Kong College of Pathologists. Opinions expressed are those of the authors or named individuals, and are not necessarily those of the Hong Kong College of Pathologists.
Dr. Calvin Yeow-Kuan Chong
Department of Pathology, Princess Margaret Hospital
Introduction
Biochemical genetics refers to the diagnosis of genetic
disorders with biochemical markers. Sir Archibald Garrod first
described the biochemical features of alkaptonuria in 19021,
and is often named as the founding father of biochemical genetics.
Throughout the past century, the practice of biochemical genetics has
evolved from spot chemical tests towards the use of chromatography and
mass spectrometry2,3. The recent
implementation of the pilot study of expanded newborn screening means,
on one hand, disorders are diagnosed earlier, and patients with such
conditions would fare better, and on the other hand, this represents an
increase in the use of confirmatory tests in biochemical genetics which
is most acutely felt at the major chemical pathology laboratories.
The screening panel of the government-initiated pilot study
included 8 aminoacidopathies, 7 organic acidurias, 6 fatty acid
oxidation defects, and 3 other disorders4.
Apart from the three disorders which required separate measurements,
all three other major classes of disorders (viz. the aminoacidopathies,
organic acidurias, and fatty acid oxidation defects) are screened by
the use of tandem mass spectrometric measurement of succinylacetone,
amino acids and acylcarnitines, all done within a period of around two
minutes.
Table 1 lists the confirmatory markers and tests for the
screened conditions 5,6: as can be seen, plasma
amino acids, urine organic acids, and plasma acylcarnitines represents
the main bulk work as confirmatory tests for these conditions. The
present article aims therefore to explore the contemporary techniques
available for these three major confirmatory tests in biochemical
genetics and attempts to identify the approaches a laboratory may seek
in order to cater for the increased demands.
Table 1. Confirmatory markers and tests for conditions covered in the government-initiated pilot study of expanded newborn screening. Specialized tests not discussed in the present article are italicized.
Amino acids
Quantitative analysis of amino acids in plasma is the first-line confirmatory test for most aminoacidopathies. Amino acids are characterized by the presence of primary amine and carboxylic acid groups in one molecule, though some imino acids, namely proline and hydroxyproline, containing an imino (a functional group containing carbon-nitrogen double bond) as well as a carboxylic acid groups are also considered under the umbrella term amino acids in medical parlance and this use of terminology is adopted in the present article.
The analytical difficulties for amino acids are obvious when one examine their chemical structures: they are amphoteric, very small (glycine has a molecular mass of 75.067 g/mol), and most of them do not possess conjugated double bonds which gives absorbance in the ultraviolet spectra7. The first and second characteristics make separation difficult when reverse phase chromatography is used, whereas the last two give rise to problems in mass spectrometric detection and ultraviolet detection respectively. There are three major methods commonly used in clinical laboratories: amino acid analysers, high performance liquid chromatography (HPLC) with pre-column derivatization, and liquid chromatography-tandem mass spectrometry (LC-MS/MS). Irrespective of the methods employed, protein precipitation is necessary before analysis.
Amino acid analysers
Amino acid analysers (AAAs) are standalone machines that employ cation-exchange chromatography using a lithium buffer system, with post-column derivatization with ninhydrin (at some 120-135 degree Celsius) and monitoring at two wavelengths8. It is considered the gold standard method for amino acid analysis. This method overcomes the difficulty in separation of amino acids by utilizing ion-exchange chromatography which relies on the charge of a molecule at a particular pH rather than the hydrophobicity and steric interactions of a molecule and that of detection by formation of purple complexes which absorb strongly at 570 nm for primary amino acids, and yellow complexes which absorb strongly at 440 nm for proline and hydroxyproline9,10. When compared with other methods, AAAs provides higher degree of automation and require less expertise from the laboratory; the major issues with AAAs are the lengthy analytical run (120 minutes is common), the cost of the analytical instruments and the proprietary nature of the reagents. Analytical interference in AAAs are rare but do occur with dipeptides, which occur in prolidase deficiency8,11, and aspartylglucosamine, which occur in aspartylglucosaminuria8, can be spotted by the 570/440 absorbance ratio.
HPLC and LC-MS/MS methods
HPLC coupled with pre-column derivatization is used in the author’s laboratory for PAA determination. The method relied on the pre-column (immediately before injection) derivatization of amino acids by orthophthalaldehyde (OPA) and 3-mercaptopropionic acid (MPA), followed by reversed phase chromatography and ultraviolet detection12-14. In the presence of a thiol reagent, OPA forms a fluorescent derivative with primary amino acids with peak absorbance/excitation at 340 nm and emission wavelength at 450 nm7,8. The major drawback of this method is the inability to detect proline (and hydroxyproline) as OPA does not react with imino acids.
A newer derivatization reagent, AccQ-Tag (6-aminoquinolyl-N-hydroxysuccinimidyl carbamate), which converts both primary and secondary amino acids into stable fluorescent derivatives, has been used with ultra-high performance liquid chromatography with ultraviolet detection15. This derivatization reagent has the advantages of covering both primary and secondary amino acids and as the derivatization products are stable, advanced autosamplers which can pipet reagent from one vial to another is not required16. Complete chemistry kit-sets that use this derivatization reagent is commercially available17.
Liquid chromatography coupled with tandem mass spectrometry is also used locally for PAA determination. As these methods are often based on reversed phase chromatography, despite the use of mass spectrometric detector, derivatization is still often employed7. A method based on the proprietary derivatization reagent AccQ-Tag has been recently described in the literature with a run-time of 6 minutes18. Isotopic internal standards are required for LC-MS/MS-based methods and it is important to note that the impracticality of having isotopic internal standards for each and every analyte would mean that the robustness of the assay is considerably weaker than optical-based methods19.
Compared with methods based on optical detection, LC-MS/MS methods had a shorter analytical runtime and better specificity. This is counterweighed by the higher capital and maintenance cost of acquiring an LC-MS/MS (and not to mention the cost of having a backup LC-MS/MS system), as well as the technical expertise necessary to operate and troubleshoot an LC-MS/MS.
Choice of method and the future
The question is different depending on the volume of testing as well as equipment availability. For laboratories that has existing HPLC-DAD equipment employing OPA derivatization there may be little incentive in adopting a new procedure: there is probably little competition for HPLC analyser time, and the inability to detect proline may not be a major issue as hyperprolinaemia type I is benign whereas hyperprolinaemia type II is classically diagnosed by the presence of N-(Pyrrole-2-carboxyl)-glycine in urine organic acids20.
On the other hand, for a laboratory seeking to provide amino acids analysis for the first time, the use of stable derivatization reagent such as AccQ-Tag mentioned above has the advantage of not requiring higher-end liquid chromatographs and the availability of commercial kits means that development time is reduced. For a laboratory anticipating a high workload, a dedicated liquid chromatograph with UV detection appears to be the simplest solution as it is robust and inexpensive. On the other hand, for laboratories with lower service demand, mass spectrometric detection may in fact be more feasible as the notion of spare LC-MS/MS capacity means that the major downside of LC-MS/MS detection, viz capital cost and technical expertise, are sunk cost to the laboratory.
Organic acids
Organic acidurias are diagnosed most commonly by urine organic acid analysis with gas chromatography-mass spectrometry (GC-MS)21, and this technique is used by many local hospitals. Though the term organic acids refers to organic compounds with a carboxylic acid group, a broader spectrum of compounds, such as uracil and xanthine, are detected in practice. Urine organic analysis is usually qualitative though quantitative analyses of some compounds (e.g. orotic acid, methylmalonic acid) are often offered.GC-MS
based urine organic acid analysis
For GC-MS analysis of urine organic acids, a creatinine-corrected amount of urine is subject to liquid-liquid extraction with ethyl acetate after acidification using hydrochloric acid, the organic extract is then dried and derivatized by N,O-bis-tri(methylsilyl)trifluoroacetamide (BSTFA) with 1% trimethylchlorosilane (TMCS)22. The resultant product is injected to the GC-MS operating in scan mode. For reliable detection of ketones, oximation with hydroxylamine hydrochloride can be performed prior to acidic extraction23.
An alternative way of performing urine organic acid analysis is to bypass the extraction step. As no acidic extraction step is done, a limited panel of amino acids, purine, pyrimidines, and mono- and di-saccharides can be detected in the same analytical run. This method has been in-use in the author’s laboratory in the past 2 decades24,25, and allows the detection of a much wider range of metabolites in urine. With no extraction, the chromatograms are extremely complex due to co-elution of analytes and therefore this approach requires expertise in post-analytical data processing to be viable as a routine method.
The interpretation of GC-MS data for urine organic acid analysis is commonly based on examination of the chromatogram (Figure 1), followed by a combination of peak integration in the total ion chromatogram followed by library searching and examination of extracted ion chromatogram at particular retention times for analytes which gives lower responses (Figure 2). The raw analyte response is then compared to locally-established age-specific reference intervals which allow clinical interpretation23.
Figure 1. Total ion chromatogram of a urine sample from a patient with malonic acidemia circulated in the ERNDIM qualitative organic acid program in 2016. The two abnormal peaks are indicated by asterisks: the first peak represents malonic acid (di-TMS derivative) and the second peak represents methylmalonic acid (di-TMS derivative).
Figure 2. Extracted ion chromatogram showing the quantifier ions (m/z 375, for aconitic acid in red; and m/z 254, for orotic acid, in black) and qualifier ions (m/z 285, for aconitic acid in green; and m/z 357, for orotic acid, in blue). They are co-eluting in many GC-MS based urine organic acid assays.
LC-MS
based urine organic acid analysis
In the recent years, liquid chromatography-mass spectrometry-based methods have been published for analysis of urine organic acids. The benefit of liquid chromatography-mass spectrometry is clear: there is no need for the cumbersome derivatization step, and heat-labile analytes can be detected26,27. The major drawbacks of LC-MS based methods are the poorer separation of analytes and higher susceptibility towards ion suppression, as electrospray ionization is much less robust against matrix effects compared to electron ionization as used in GC-MS based methods28. The difficulty of developing an in-house LC-MS based method for urine organic acids lies in the procurement of a practically endless list of organic acid standards to establish the retention time and multiple reaction monitoring ratios. At the time of writing, there is at least one commercial kit that has been made available (Zivak Organic Acids LC-MS/MS analysis kit), but this commercial kit did not utilize isotopic internal standards even for critical analytes (such as hexanoylglycine and orotic acid) and one may wish to validate extensively the robustness of such assays against matrix effects.
Choice
of method and the future
Out of the three assays discussed in the present article,
urine organic acid analysis represents the most difficult of the three
to establish in a laboratory. There is first the difficulty in
establishing age-specific reference intervals, and then difficulty in
acquiring a large number of chemical standards. A good starting point
would be obtaining the bi-level quality controls for urine organic acid
and special assays for urine from the ERNDIM
network which consist of a
number of critical analytes. As for choice of internal
standards, while traditional choices such as tropic acid and
pentadecanoic acid are often employed, deuterated internal standards
covering critical analytes are much more available nowadays (e.g.
methylmalonic acid-d3 and orotic acid-1,3-15N2) and their addition may
improve quantitation of these critical analytes, the former being
commonly quantified and the latter being prone to analytical errors29.
For laboratories with existing GC-MS based urine organic acid assay, the question is probably whether to adopt LC-MS based solution. The belief that organic aciduria always results in sky-high level of abnormal metabolites in urine is flawed: the low-excretor phenotype of glutaric aciduria type I can serve as the classical example30. The difficulty may be mitigated somewhat if acylcarnitines and acylglycines are measured by the LC-MS based assays as glutarylcarnitine has been shown to be informative even for low-excretor GA I patients31. Overall, it remains to be seen whether LC-MS based organic acid analysis could stand alone replacing, rather than complementing, GC-MS based assays.
Free carnitine and acylcarnitines
Quantitative analysis of free carnitine and acylcarnitines in plasma represents the first-line confirmatory test for fatty acid oxidation disorders. Analysis of free carnitine with calculation of fraction of excretion can be helpful in the diagnosis of carnitine uptake defect, as is measurement of urine glutarylcarnitine for the diagnosis of glutaric aciduria type I as discussed above31. Carnitine is a quaternary ammonium compound with a carboxylic acid group, as well as a hydroxyl group with which acyl groups are attached to form acylcarnitines; as such, it forms zwitterions under physiological pH. Derivatization to esters by incubation with an alcohol (typically butanol) has been employed32 though locally underivatized analysis at acidic pH is commonly used33.
For underivatized analysis, plasma is first diluted with a mixture of isotopic internal standards, and acidified acetonitrile is slowly added to precipitate plasma proteins. The sample is vortexed, centrifuged, followed by evaporation of the supernatant to dryness and reconstituted for analysis by positive electrospray ionization-tandem mass spectrometry with or without liquid chromatography separation. Data can be collected in multiple reaction monitoring (MRM) mode, or in precursor ion scan with accumulation of ions, commonly known as multichannel acquisition (MCA) mode34 (Figure 3). The American College of Medical Genetics Guideline, published in 2008, suggested the use of precursor ion scan as it permitted the evaluation of the whole acylcarnitine profile, as well as the detection of drug artefacts, interfering compounds and assessment of derivatization35.
Figure 3. Cumulative precursor scan mass spectrum for a blood-spot sample distributed in the ERNDIM Qualitative Acylcarnitine Program in 2014. In this specimen, elevated signals at m/z ratio 260 (C6-carnitine), 288 (C8-carnitine), and 314 (C10:1-carnitine) can be seen. The pattern would be compatible with MCAD deficiency. (Mass spectra courtesy of Mr CK Lai, Chemical Pathology Laboratory, PMH)
Derivatization and chromatographic separation
In the analysis of carnitine and acylcarnitines, butyl-ester derivatization enhances the formation of positively-charged ion by reacting with carboxylic groups, and causes mass separation of dicarboxylic-carnitines and hydroxy-acylcarnitines (e.g. C4DC-carnitine and C5OH-carnitine), which are isobaric when underivatized36. Derivatization is typically performed at highly acidic conditions (e.g. 3N hydrochloric acid at 65°C for 15 minutes)35. On the other hand, chromatographic separation allows the separate determination of individual isomeric constituents (e.g. C4DC-carnitines include succinylcarnitine and methylmalonylcarnitine; and C5OH-carnitines include 3-hydroxyisovalerylcarnitine and 2-methyl-3-hydroxybutyrylcarnitine) (Figure 4). With meticulous chromatographic separation, most biologically relevant isomeric species could be separately quantified37.
Choice
of method and the future
The question for the laboratory is, first, whether to employ
the derivatization procedure. The advantage of derivatization is the
mass separation of hydroxylacylcarnitines and carnitine derivatives of
dicarboxylic acids; the problems associated with derivatization are the
partial hydrolysis of acylcarnitines because of the high temperature
and strongly acidic condition employed33. The
other considerations
are whether to employ chromatography and if so, how extensive should it
be: it would then be a delicate balance between through-put, diagnostic
specificity, and analyser-time that is available. With the improvement
of separation capability of liquid chromatographs and sensitivity of
modern mass spectrometers, it is suggested that a short UHPLC program
combined with both scheduled MRM and precursor ion scan function would
be a good compromise.
Figure 4. SRM chromatogram of m/z 262>85 showing chromatographic separation of different species of isobaric (C4DC/C5OH) acylcarnitines (viz. succinylcarnitine at 4.68 minutes, two diastereomeric peaks of methylmalonylcarnitine at 5.2 and 5.35 minutes, and 3-hydroxyisovalerylcarnitine at 5.82 minutes) could be individually identified and quantified with liquid chromatography-tandem mass spectrometry without derivatization. (a) sample with normal amounts of succinylcarnitine; (b) sample with abnormal amounts of methylmalonylcarnitines (Chromatograms courtesy of Mr CK Lai, Chemical Pathology Laboratory, PMH)
Conclusions
The three assays discussed above represent the bulk of workload for most metabolic laboratories. The planned implementation of universal expanded newborn screening in Hong Kong means that the demand would increase, and the phenotype will be less defined, as tests are requested for patients who may not yet present with features of an inborn error and importantly, patients with only borderline elevation of analytes.
From a Bayesian point of view, this change in pre-test probability would mean that, if the analytical quality and interpretative capacity remains the same (which affect the likelihood ratio of positive results), the post-test probability would suffer from a negative impact. The quest for the metabolic bench of any major pathology laboratory is then to improve both throughput and quality of analysis at the same time, an impossible task, as the Duke of Norfolk wrote in 1538, “a man can not have his cake and eat his cake”38. The technology improvements as reviewed in the present article may aid in the analytical quality but the quest for improved interpretative capacity remains on the training and education of our present and coming generations of pathologists.
Reference
-
Garrod, AE. The incidence of alkaptonuria: a study in chemical individuality. The Lancet. 1902 Dec 13;160(4137):1616–20.
-
Millington DS, Kodo N, Norwood DL, Roe CR. Tandem mass spectrometry: a new method for acylcarnitine profiling with potential for neonatal screening for inborn errors of metabolism. Journal of inherited metabolic disease. 1990;13(3):321–4.
-
Schulze A, Lindner M, Kohlmüller D, Olgemöller K, Mayatepek E, Hoffmann GF. Expanded newborn screening for inborn errors of metabolism by electrospray ionization-tandem mass spectrometry: results, outcome, and implications. Pediatrics. 2003;111(6):1399–406.
-
Mak, CM. Newborn Screening: Past, Present and the Future. Topical Update, The Hong Kong College of Pathologists. 2016;11(2):1–10.
-
Ozben T. Expanded newborn screening and confirmatory follow-up testing for inborn errors of metabolism detected by tandem mass spectrometry. Clinical Chemistry and Laboratory Medicine. 2012;51(1):157–176.
-
Saudubray J-M, Berghe G van den, Walter JH. Inborn Metabolic Diseases. Springer-Verlag Berlin Heidelberg; 2012.
-
Kaspar H, Dettmer K, Gronwald W, Oefner PJ. Advances in amino acid analysis. Anal Bioanal Chem. 2009 Jan;393(2):445–52.
-
Duran M. Amino Acids. In: Blau N, Duran M, Gibson KM, editors. Laboratory Guide to the Methods in Biochemical Genetics [Internet]. Berlin, Heidelberg: Springer Berlin Heidelberg; 2008 [cited 2018 Nov 24]. p. 53–89. Available here.
-
Friedman M, Williams LD. Stoichiometry of formation of Ruhemann’s purple in the ninhydrin reaction. Bioorganic Chemistry. 1974;3(3):267–80.
-
Cunico RL, Schlabach T. Comparison of ninhydrin and o-phthalaldehyde post-column detection techniques for high-performance liquid chromatography of free amino acids. Journal of Chromatography A. 1983;266:461–70.
-
Ferreira CR, Cusmano-Ozog K. Spurious Elevation of Multiple Urine Amino Acids by Ion-Exchange Chromatography in Patients with Prolidase Deficiency. JIMD Rep. 2016 Apr 12;31:45–9.
-
Fürst P, Pollack L, Graser TA, Godel H, Stehle P. Appraisal of four pre-column derivatization methods for the high-performance liquid chromatographic determination of free amino acids in biological materials. Journal of Chromatography A. 1990;499:557–69.
-
Schuster R. Determination of amino acids in biological, pharmaceutical, plant and food samples by automated precolumn derivatization and high-performance liquid chromatography. Journal of Chromatography B: Biomedical Sciences and Applications. 1988;431:271–84.
-
Terrlink T, Van Leeuwen PA, Houdijk A. Plasma amino acids determined by liquid chromatography within 17 minutes. Clinical chemistry. 1994;40(2):245–9.
-
Narayan SB, Ditewig-Meyers G, Graham KS, Scott R, Bennett MJ. Measurement of plasma amino acids by Ultraperformance® Liquid Chromatography. Clinical Chemistry and Laboratory Medicine. 2011;49(7):1177–1185.
-
van Wandelen C, Cohen SA. Using quaternary high-performance liquid chromatography eluent systems for separating 6-aminoquinolyl-N-hydroxysuccinimidyl carbamate-derivatized amino acid mixtures. Journal of Chromatography A. 1997;763(1–2):11–22.
-
Armenta JM, Cortes DF, Pisciotta JM, Shuman JL, Blakeslee K, Rasoloson D, et al. A sensitive and rapid method for amino acid quantitation in malaria biological samples using AccQ•Tag UPLC-ESI-MS/MS with multiple reaction monitoring. Anal Chem. 2010 Jan 15;82(2):548–58.
-
Gray N, Zia R, King A, Patel VC, Wendon J, McPhail MJW, et al. High-Speed Quantitative UPLC-MS Analysis of Multiple Amines in Human Plasma and Serum via Precolumn Derivatization with 6-Aminoquinolyl-N-hydroxysuccinimidyl Carbamate: Application to Acetaminophen-Induced Liver Failure. Anal Chem. 2017 Feb 21;89(4):2478–87.
-
Sharer JD, Biase ID, Matern D, Young S, Bennett MJ, Tolun AA. Laboratory analysis of amino acids, 2018 revision: a technical standard of the American College of Medical Genetics and Genomics (ACMG). Genetics in Medicine. 2018 Oct 19;1.
-
Walker V, Mills GA. N-(pyrrole-2-carboxyl) glycine a diagnostic marker of hyperprolinaemia type II: mass spectra of trimethylsilyl derivatives. Clin Chim Acta. 2009 Jul;405(1–2):153–4.
-
Christou C, Gika HG, Raikos N, Theodoridis G. GC-MS analysis of organic acids in human urine in clinical settings: a study of derivatization and other analytical parameters. Journal of Chromatography B. 2014;964:195–201.
-
Rinaldo P. Organic Acids. In: Blau N, Duran M, Gibson KM, editors. Laboratory Guide to the Methods in Biochemical Genetics [Internet]. Berlin, Heidelberg: Springer Berlin Heidelberg; 2008 [cited 2018 Nov 30]. p. 137–69. Available here.
-
Gallagher RC, Pollard L, Scott AI, Huguenin S, Goodman S, Sun Q, et al. Laboratory analysis of organic acids, 2018 update: a technical standard of the American College of Medical Genetics and Genomics (ACMG). Genet Med. 2018;20(7):683–91.
-
Lee H-CH, Lai C-K, Yau K-CE, Siu T-S, Mak CM, Yuen Y-P, et al. Non-invasive urinary screening for aromatic l-amino acid decarboxylase deficiency in high-prevalence areas: A pilot study. Clinica Chimica Acta. 2012 Jan 18;413(1):126–30.
-
Au KM, Lai CK, Yuen YP. Diagnosis of dihydropyrimidine dehydrogenase deficiency in a neonate with thymine-uraciluria. Hong Kong Medical Journal. 2003;9(2):130–3.
-
Körver-Keularts IMLW, Wang P, Waterval HWAH, Kluijtmans LAJ, Wevers RA, Langhans C-D, et al. Fast and accurate quantitative organic acid analysis with LC-QTOF/MS facilitates screening of patients for inborn errors of metabolism. J Inherit Metab Dis. 2018 May 1;41(3):415–24.
-
Yassine MM, Dabek-Zlotorzynska E. Application of ultrahigh-performance liquid chromatography–quadrupole time-of-flight mass spectrometry for the characterization of organic aerosol: Searching for naphthenic acids. Journal of Chromatography A. 2017 Aug 25;1512:22–33.
-
Taylor PJ. Matrix effects: the Achilles heel of quantitative high-performance liquid chromatography–electrospray–tandem mass spectrometry. Clinical biochemistry. 2005;38(4):328–34.
-
Scott C, Langhans C-D, Roux C. Qualitative Organic Acid. In: ERNDIM Workshop. Manchester, UK; 2017.
-
Kölker S, Christensen E, Leonard JV, Greenberg CR, Boneh A, Burlina AB, et al. Diagnosis and management of glutaric aciduria type I–revised recommendations. Journal of inherited metabolic disease. 2011;34(3):677.
-
Tortorelli S, Hahn SH, Cowan TM, Brewster TG, Rinaldo P, Matern D. The urinary excretion of glutarylcarnitine is an informative tool in the biochemical diagnosis of glutaric acidemia type I. Molecular Genetics and Metabolism. 2005 Feb 1;84(2):137–43.
-
Dhillon KS, Bhandal AS, Aznar CP, Lorey FW, Neogi P. Improved tandem mass spectrometry (MS/MS) derivatized method for the detection of tyrosinemia type I, amino acids and acylcarnitine disorders using a single extraction process. Clinica Chimica Acta. 2011;412(11–12):873–9.
-
Ho CS, Cheng BSS, Lam CWK. Rapid Liquid Chromatography–Electrospray Tandem Mass Spectrometry Method for Serum Free and Total Carnitine. Clinical Chemistry. 2003 Jul 1;49(7):1189–91.
-
Matern D. Acylcarnitines, Including In Vitro Loading Tests. In: Blau N, Duran M, Gibson KM, editors. Laboratory Guide to the Methods in Biochemical Genetics [Internet]. Berlin, Heidelberg: Springer Berlin Heidelberg; 2008 [cited 2018 Nov 30]. p. 171–206. Available here.
-
Rinaldo P, Cowan TM, Matern D. Acylcarnitine profile analysis. Genetics in Medicine. 2008 Feb;10(2):151–6.
-
Vreken P, Van Lint AEM, Bootsma AH, Overmars H, Wanders RJA, Van Gennip AH. Quantitative plasma acylcarnitine analysis using electrospray tandem mass spectrometry for the diagnosis of organic acidaemias and fatty acid oxidation defects. Journal of inherited metabolic disease. 1999;22(3):302–6.
-
Giesbertz P, Ecker J, Haag A, Spanier B, Daniel H. An LC-MS/MS method to quantify acylcarnitine species including isomeric and odd-numbered forms in plasma and tissues. Journal of lipid research. 2015;jlr. D061721.
-
Letters and Papers, Foreign and Domestic, Henry VIII, Volume 13 Part 1, January-July 1538 [Internet]. 1892. Available here.
Abbreviations:
Plasma amino acids (PAA):
Arginine (Arg), Citrulline (Cit), Homocystine (Hcy), Isoleucine (Ileu), Leucine (Leu), Methionine (Met), Ornithine (Orn), Phenylalanine (Phe), Threonine (Thr), Tyrosine (Tyr), Valine (Val).
Urine organic acids (UOA):
ethylmalonic acid (EMA), glutaric acid (GA), 2-hydroxyglutaric acid (2-OHGA), 3-hydroxyglutaric acid (3-OHGA), 3-methylglutaric acid (3-MGA), 3-methylglutaconic acid (3-MGCA), hexanoylglycine (HG), 3-hydroxyisovaleric acid (3-OHIVA), Isovalerylglycine (IVG), 3-methylcrotonylglycine (3-MCG), Methylcitric acid (MCA), Methylmalonic acid (MMA), 3-hydroxypropionic acid (3-OHPA), propionylglycine (PG), phenylpropionylglycine (PPG), Tiglylglycine (TG).
Plasma acylcarnitines (PAC):
Free carnitine (C0).
Others:
6-pyruvoyl-tetrahydropterin synthase (PTPS), 3-hydroxy-3-methylglutaryl CoA (HMG-CoA), Carnitine-acylcarnitine translocase (CACT), Carnitine palmitoyltransferase II (CPT-II), Medium-chain acyl-CoA dehydrogenase (MCAD), Very long-chain acyl-coA dehydrogenase (VLCAD).